By Sanjay Asrani, MD; Lylas G. Mogk, MD, and Mary Lou Jackson, MD; Zélia M. Corrêa, MD, PhD; and Mehdi Roozbahani, MD, and J. Bradley Randleman, MD
Download PDF
Because the end of the year is a fitting time to take stock of recent events, EyeNet has asked a few of its board members to review developments or trends in their areas of expertise and to consider which of these has the greatest potential to shape their subspecialty over the next several years. Interestingly, many of their observations touched on—or focused squarely on—technologies or testing that may offer personalized patient care. Below are their perspectives in the fields of glaucoma, low vision rehabilitation, ocular oncology, and refractive surgery and corneal ectatic disease.
DR. ASRANI ON GLAUCOMA
Where Glaucoma Is Now, and Where It Is Going: A Survey
Glaucoma diagnosis and management are both complex issues. This is because glaucoma is a group of diseases, not all of which are associated with raised intraocular pressure (IOP). Today, diagnosis is made by a pattern recognition of a typical optic nerve appearance and/or a typical visual field defect. Visual field testing is problematic, however, because it is universally disliked by patients, and its fluctuating and subjective nature makes interpretation difficult for the physician. Our one objective technique of diagnosis involves optical coherence tomography (OCT), which also has significant pitfalls, such as artifacts, relying on the OCT report, using it in isolation, etc. To state the obvious, there is room for improvement. Fortunately, new areas of innovation and investigation are opening up.
Objective assessments. The next frontier in diagnostics is in detecting glaucoma progression objectively. Many studies in progress involve using OCT early enough to initiate or advance treatment before visual field loss occurs or worsens. New technology for objectively measuring visual fields is being refined using head-mounted sets and virtual reality right here at Duke University. (See “The New World of Virtual Reality,” in the October EyeNet.) And the promise of DARC technology (Detection of Apoptosing Retinal Cells) using annexins to label dying/stressed ganglion cells lies in being able to detect the IOP level that is detrimental to that particular eye and possibly developing drugs or rescue mechanisms to halt such a process.1 This technology is now being tested in clinical trials. Farther on the horizon, there is a possible future in detecting stressed ganglion cells by detecting flavoprotein fluorescence in the retina.2
Home monitoring. With respect to IOP measurement, the home ICare (rebound tonometry) device has the potential for collecting a lot more data in the patient’s own environment than has been previously possible. Additionally, implantable devices to measure and relay IOP remotely are under development. Availability of such data would have the same impact as home glucose monitoring devices have had on the management of diabetes.
New drugs. Over the last year, the field of glaucoma has had 2 new drug approved, Vyzulta and Rhopressa, and a fixed combination of latanoprost and netarsudil is under FDA review. Much-anticipated sustained drug delivery systems are likely to be available soon, addressing the dry eye and daily compliance issues that plague glaucoma patients. Various modalities are under investigation: intraocular implants, subconjunctival implants, cul-de-sac, and punctal implants, among others. It is yet to be established whether such modalities will help with long-term, consistent reduction of IOP and IOP fluctuations; keep the need for removal or replacement to a minimum; and gain patient acceptance, especially for more invasive models needed for chronic disease.
Surgery. Management of glaucoma has, thankfully, involved many new treatment modalities over the last 5 years in the form of minimally invasive glaucoma surgeries (MIGS). These devices are evolving to become options for stages other than mild glaucoma. Newer and more biocompatible devices (e.g., Hydrus, InnFocus microshunt, iStent) are awaiting approval.
There is a need for a surgery that is as effective as a well-functioning trabeculectomy but that has a more predictable postoperative and long-term course. Achieving such a goal may require harnessing the mechanisms of action of 2 (or more) devices. The limitation will lie in reimbursement issues when more than 1 MIGS device is implanted simultaneously.
New therapies. Another frontier in glaucoma treatment is the repopulation of degenerated trabecular beams with healthy trabecular meshwork cells either using stem cells or cell transplants.3 This may permit reactivation of the patient’s own trabecular meshwork to maintain IOP homeostasis. Another promising treatment is described in the recently published studies on the ability of nicotinamide to reverse or halt mitochondrial death in axons in animal models of glaucoma.4
Challenges in glaucoma abound. However, we are fortunate that in the last 5-7 years, there have been many promising developments in the field. It is also encouraging that the brightest of minds are applying their expertise toward the understanding and management of glaucoma. In addition, there is more interest in glaucoma in the device and pharmaceutical industry. The future of glaucoma management is very bright.
___________________________
1 Yap TE et al. Cells. 2018;7(6).
2 Geyman LS et al. J Glaucoma. 2018;27(7):592-599.
3 Snider EJ et al. Sci Rep. 2018;8(1):12251.
4 Williams PA et al. J Glaucoma. 2017;26(12):1161-1168.
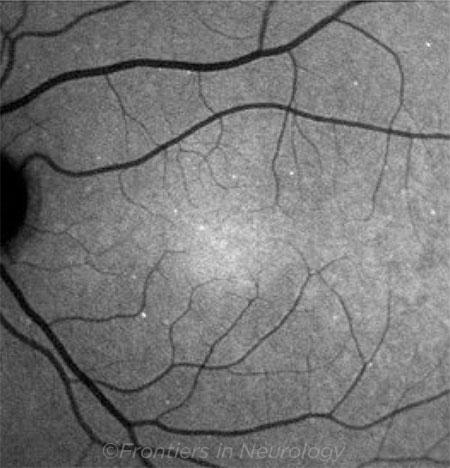 |
DARC. Detection of apoptosing retinal cell count in a glaucoma patient. Compared with healthy patients, DARC counts are increased in affected glaucoma patients. Copyright © 2018 Yang, Al-Mugheiry, Normando, and Cordeiro. This image was published in is an open-access article distributed under the terms of the Creative Commons Attribution License (CC BY). The use, distribution, or reproduction in other forums is permitted, provided the original author(s) and the copyright owner are credited and that the original publication in this journal is cited, in accordance with accepted academic practice. No use, distribution, or reproduction is permitted which does not comply with these terms. This image originally appeared in Yang E et al. Frontiers in Neurology. 2018;9:1-5, Article 338.
|
DR. MOGK AND DR. JACKSON ON LOW VISION
Vision Rehabilitation Is New and on the Rise
For the last half of the 20th century, the focus in vision rehabilitation was on blind skills, including braille and long cane training, and on education for children and job training for adults. Optometrists dispensed magnifiers and telescopes, and the U.S. Department of Veterans Affairs, state governments, private agencies, and intermediate school districts offered rehabilitation services to legally blind adults and visually impaired children and youth.
This system worked well until the late ’90s, when seniors with age-related macular degeneration (AMD) became the largest segment of the visually impaired population. Their needs, skills, and learning modes were very different from those of blind children and adults. Additionally, their central vision loss demanded intervention as soon as reading and driving were compromised, well before reaching legal blindness at 20/200. As a result, there was a mismatch between needs and available public services.
This inspired the quest among ophthalmologists and low vision ODs to gain Medicare coverage for vision rehabilitation for seniors who were not yet legally blind, and it inspired specialty training for occupational therapists (OTs), whose skill set includes dealing with the many comorbidities common to seniors.
A New and Expanding Specialty
Medicare coverage for vision rehabilitation became available nationally in 2003, making it economically feasible for group practices and academic ophthalmology programs to offer this critical service, with an MD or OD performing the low vision exam and OTs conducting rehabilitation training. Programs in academic departments continue to grow, both in number and size. Specialty training in vision rehabilitation for OTs is thriving, with The University of Alabama in Birmingham, Salus University in Pennsylvania, and Western Michigan University offering advanced certificates for OTs. Many private agencies are now adding OTs to their staffs.
For awhile now, knowledge has been spreading beyond the low vision community. For example, the Academy has published a Focal Points, a Preferred Practice Pattern, and a coding module in vision rehabilitation. The Basic Clinical and Science Course series includes a newly revised chapter, and the ONE Network offers tutorials in vision rehabilitation. The Academy annual meeting includes symposia, breakfast with the experts, and instruction courses in vision rehabilitation, sponsored by the Vision Rehabilitation Committee, chaired by John D. Shepherd, MD.
Beyond that, an increasing number of academic programs are requesting Grand Rounds speakers in vision rehabilitation. Internationally, among its Priority Assistive Products List, the World Health Organization has included 9 low vision devices; it has published the International Consensus Document on Vision Rehabilitation Standards; and it is about to publish a vision rehabilitation curriculum for training personnel. Vision rehabilitation has come a long way in a short time.
Technology
Not surprisingly, technology has been a cornerstone of contemporary vision rehabilitation. The original breakthrough technologies included video cameras with screens (closed-circuit TV or video magnifiers). These technologies continue to be updated and are a mainstay for those with low vision. Talking devices, including watches, scales, timers, and liquid level indicators, have been available for years.
Technology for all vision. The big news in technology is that many of the most important devices for patients with vision loss are the same devices used by the sighted population: smartphones, tablets, and computers. This makes them readily available and psychologically comfortable.
- Computers, of course, are endlessly adaptable, with built-in accessibility features and software that can enlarge text or offer audio input and output.
- E-readers, like Kindle, offer large print sizes and reverse polarity (white text on a black background). The latter is particularly helpful to the many low vision patients with decreased contrast sensitivity. Tablets offer magnification, text to speech, voice recognition, and photography without requiring precise focus.
- Smartphones include built-in powerful flashlights, magnification features, cameras, and voice recognition such as Siri, in addition to a bevy of apps that read text aloud, interpret bar codes, identify colors, read handwriting, and orally describe objects and people. These include, among others, TapTapSee for all smartphones, Seeing AI for iOS at no charge, and the KNFB Reader for a fee.
- Smart speakers (e.g., Alexa, Google Home, or Echo) offer easy audio access to weather, news, music, and information for everyone.
- Ride services, including Uber and Lyft, offer welcome travel options in the many places throughout this country with limited public transportation. Uber has instituted a “Go Go Granny” service that allows riders with low vision to phone in their description along with their pickup location, so the driver can identify them.
- Fully autonomous vehicles will someday be a game changer for individuals with low vision. Currently, partially autonomous vehicles requiring a capable driver are in development.
Tech for impaired vision. Technologies designed specifically for those with visual impairments are bountiful and include but are not limited to the following:
- Head-mounted devices for stationary use that magnify at varying distances offer a broad, clear view rather than the limited area viewed through a magnifier or telescope. These include, among others, the IrisVision, NuEyes, and E-Sight, each with its particular features and price points.
- The OrCam is a camera mounted on an eyeglass frame that offers text to speech capability, as well as audio descriptions of objects and people. It is for stationary or mobile use.
- A subscription service, called AIRA, connects the person with vision loss to a live agent via wearable smart glasses. The agent can see the user’s environment in real time, knows their location by GPS, and can assist with navigation, finding objects, or doing tasks.
- For individuals with significant visual field loss and mobility issues, GPS in smartphones (e.g., Blindsquare) or as wearable systems that vibrate (iWatch) are available to assist with way finding.
- Even white canes have become high tech. The SmartCane has ultrasound to detect objects. Other detection devices can be clipped on clothing (BuzzClip) or worn as glasses (iGlasses).
- Smaller-scale technologies include audio medication labels (ScriptTalk) and Digital Accessible Information System (DAISY) with over 600,000 free/low cost formatted audiobooks. Optical character recognition technology has made freestanding text-to-speech scanning devices possible (e.g., the Sara), which reads printed material aloud.
Further down the road—but currently available to certain individuals who have total or near total blindness—is artificial vision, being developed in centers around the world. The most advanced example of this is the Argus II Second Sight retinal prosthesis.
Conclusion
Vision rehabilitation is a vibrant, growing specialty. Point in fact, the Academy has declared referral to vision rehabilitation as the standard of care in ophthalmology. The more low vision programs that there are, the greater the benefit to patients and the greater the likelihood of those services being of high quality. Advances in technology, coupled with training to address the spectrum of daily activities, promise to change the experience and lighten the burden of visual impairment.
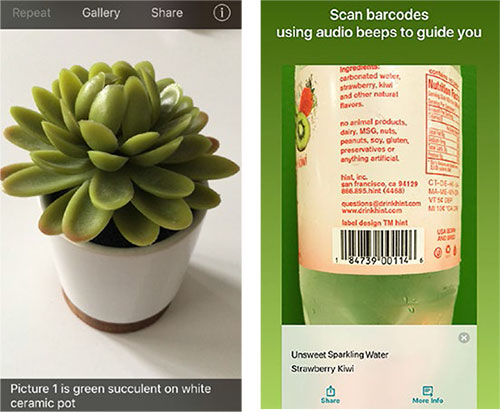 |
LOW VISION APPS. With TapTapSee (left), the user takes a photo or video, and the app identifies the object aloud. Seeing AI (right) offers multiple “channels” that can read documents and identify objects, people, and scenes.
|
DR. CORRÊA ON OCULAR ONCOLOGY
Trending Toward Personalized Medicine
There is a lot of talk about personalized, or precision, medicine today. This is thanks to the exciting possibility of using available knowledge in molecular and cell biology, genetics, and genomics to evaluate each patient. Such information may help physicians with prevention, screening, and treatment strategies that may be more effective than those that are currently available. Another goal with personalized medicine is to find treatments with fewer side effects compared to standard options. Ultimately, by performing genetic tests on cancer cells and on normal cells, physicians may be able to customize treatment to each patient’s needs.
Since completion of the human genome sequencing a little over 15 years ago, light has been shed on hundreds of genes involved in the pathogenesis of diseases. In ophthalmology, faulty genes are implicated in diseases ranging from rare hereditary syndromes to common conditions such as myopia, primary open-angle glaucoma, AMD, and ocular cancers.
Survival Prognosis in Uveal Melanoma
Uveal melanoma has probably been the biggest challenge for ocular oncologists due to its unpredictable nature and its ability to metastasize. Ample literature has been published on the clinical, histopathologic, and chromosomal features of uveal melanoma and their association with patient outcomes.1-3 Despite the fact that ocular oncologists have refined those features and studied them to exhaustion, old parameters have failed to provide a systematic understanding of this tumor’s biology.
Gene expression profiling. The identification of monosomy 3 as a risk factor for development of metastatic disease in patients with uveal melanoma occurred more than 20 years ago,4,5 but the first significant step toward more precise identification of patient risk was made with high-density microarrays to study gene expression profiles (GEP) of these tumors.6-7 Researchers showed that uveal melanomas exist in 1 of 2 basic molecular forms that are intensely associated with metastatic proclivity: class 1 tumors have a low risk and class 2 tumors have a high risk of metastasis. Using sophisticated bioinformatic analyses, studies have shown that the genes expressed in class 1 tumors are similar to those in normal uveal melanocytes (derived from the neural crest), and genes expressed in class 2 tumors resemble primitive stem-like cells.
With this new information in mind, it seems likely that previously identified histopathologic risk factors for metastasis, such as epithelioid cell type and vasculogenic mimicry patterns, correlate with de-differentiated stem-like features of these tumors.7 This is just one example of how GEP discoveries have provided new insights into the pathobiology of uveal melanoma. Subsequently, independent peer-reviewed publications have shown that the prognostic accuracy of GEP outperforms clinicopathologic features and chromosomal gains and losses.6,8
Prognostic testing. While relatively new, prognostic testing is becoming an important part of risk stratification of patients with posterior uveal melanomas. Understanding the information obtained by each test and its application is fast becoming an important part of ocular oncology clinical practice. Currently, the 2 commercially available prognostic tests for uveal melanoma employ different platforms that consequently yield distinct results that should be viewed differently.9
Impact Genetics test. One of the prognostic tests is Impact Genetics Uveal Melanoma Prognostic Genetic Test (Impact Genetics).
- How it works. The test involves multiplex ligand probe amplification to evaluate copy number on chromosomes 1p, 3, 6, and 8 to detect monosomy, disomy, and trisomy; microsatellite analysis on chromosome 3 to detect loss of a chromosome copy and isodisomy; and sequencing GNAQ, GNA11, SF3B1, and EIF1AX to detect frequently occurring mutations in uveal melanoma tumor for confirmation of tumor sampling where indicated.9
- Concerns. Before using this test, consider the following. This assay employs preferably FFPE specimens and requires larger quantities of tissue than the test below. Multiple articles describe this assay and its complex risk stratification as both challenging to apply in clinical practice and to explain to patients. There has been no prospective validation of this test. Another limitation to adopting this test: insurance coverage for patients incurring out-of-pocket costs.
Castle Biosciences test. The other test, UMDecision-Dx (Castle Biosciences), is used to detect up-regulation or down-regulation of particular genes of interest in minute tissue samples.7-9 This test’s GEP technique mainly uses fine-needle biopsy samples that are too small to be reliably assessed using chromosome-based assays. It evaluates the expression of 12 discriminating genes and 3 control genes using quantitative polymerase chain reaction (PCR) on a microfluidics platform after targeted amplification.
- Patient stratification. Through GEP assay of untreated uveal melanoma tissue samples, 2 distinct prognostic classes and 1 subdivision of these classes have been identified and shown to predict metastatic risk and strongly correlate with survival in patients with posterior uveal melanoma. Patients with class 1 tumor gene expression profiles have low-grade tumors with a decreased risk of metastatic spread. Class 1 patients have been further subdivided into A and B according to their mid-term prognosis. On the other hand, patients with class 2 tumor gene expression profiles have high-grade tumors with an increased tendency to metastasize.10
- A comparison with current testing. When compared to the presence of monosomy 3, and to clinical and pathologic tumor features, GEP demonstrated superior accuracy at predicting the risk of metastatic disease in patients with primary uveal melanoma. Importantly, because GEP is an RNA-based assay, it predicts how the tumor cells are likely to behave as far as metastatic spread, whereas DNA-based assays provide a snapshot in time of the genetic makeup of tumor cells.10 UMDecision-Dx is the only prognostic test for uveal melanoma to undergo prospective multicenter validation, which is required for a cancer biomarker to achieve the highest level I evidence according to the National Comprehensive Cancer Network Task Force on cancer biomarkers and the Tumor Marker Utility Grading System.
- PRAME is recent finding. Recently, researchers have shown that preferentially expressed antigen in melanoma (PRAME) mRNA expression was associated with an increased risk of metastasis in both class 111 and class 212 melanomas. This test has been incorporated into the GEP test and has been reported to increase the accuracy of the assay.
Although survival of uveal melanoma patients remains poor, researchers are gaining important understandings of this disease. First, they now believe that prognostic mutations occur prior to primary tumor treatment. Second, driver mutations have been investigated and 3 appear to be mutually exclusive: Class 2 profile tumors are strongly associated with inactivating mutations in the BRCA1-Associated Protein 1 (BAP1) tumor suppressor gene; EIF1AX correlates with low-risk class 1A signature tumors; and SF3B1 correlates with moderate risk class 1B tumors.12 These newly detected mutations are providing insight into the pathways that these tumors may take.
Treatment for Uveal Melanoma Metastasis
Despite these recent discoveries in prognostication, personalized medical care to treat metastatic disease is underwhelming, but promising therapies are in the pipeline.
Available therapies. Current options to treat metastatic uveal melanoma include liver-directed chemotherapy, systemic and targeted immune therapies, and targeted therapy with T cells directed against tumor-associated antigens.13 Unfortunately, these treatments have yielded long-lasting responses in very few patients. As for immunotherapy specifically, its potential efficacy has been limited, presumably owing to the small number of mutations leading to neoantigen expression in uveal melanoma; immunotherapy with checkpoint inhibitors has shown very low response rates in metastatic uveal melanoma.
Investigational therapies. A number of novel therapies based on supposed biological mechanisms are being investigated in the adjuvant setting. The growth factor receptors c-Met and c-Kit are highly expressed in uveal melanoma and may play a role in metastatic progression.
Target: c-Met. Crizotinib is a tyrosine kinase inhibitor (TKI) shown to inhibit phosphorylation of c-Met and in vitro migration of uveal melanoma cells. Interestingly, at doses that selectively inhibit c-Met, crizotinib only marginally reduced the growth of established tumors, suggesting that other tyrosine kinase receptors such as epidermal growth factor receptor (EGFR) and insulin growth factor receptor 1 (IGFR1) are critical for uveal melanoma cell proliferation and survival.
Target: c-Kit. Sunitinib—another TKI that inhibits c-Kit, vascular endothelial growth factor receptor (VEGFR), and other receptors—yielded a 5-year survival benefit (75% versus 55%) compared to matched controls in a retrospective study.
Both crizotinib and sunitinib are being evaluated in ongoing adjuvant trials [ClinicalTrials.gov identifiers: NCT02223819, NCT02068586].
Histone inhibitor. Because BAP1 has been shown to regulate melanocytic differentiation by modifying histones, researchers are looking into personalized trials using histone deacetylase (HDAC) inhibitors for class 2 tumors (BAP1 mutation).14 Based on the potential role for HDAC inhibition in the adjuvant setting, valproic acid and vorinostat are being evaluated in ongoing trials [ClinicalTrials.gov identifiers: NCT02068586, NCT01587352].
Others. Another promising targeted therapy involves the aberrant hypomethylation of PRAME that can be activated in uveal melanomas and is associated with increased metastatic risk in both GEP classes. Finally, various other strategies are being investigated including immune-based therapies such as immune checkpoint inhibition and autologous dendritic cell vaccines.
Conclusion
In summary, exciting new research is showing that the treatment of metastatic uveal melanoma will likely involve personalized management strategies based on genetic testing. As we look even further into the future, new progress in looking at the peripheral blood of melanoma patients may represent another frontier on the prognostic evaluation of these patients.
___________________________
1 Augsburger JJ, Gamel JW. Cancer. 1990;66(7):1596-1600.
2 McLean IW et al. Ophthalmology. 1995;102(7):1060-1064.
3 Gamel JW et al. Invest Ophthalmol Vis Sci. 1992;33(6):1919-1922.
4 Prescher G et al. J Natl Cancer Inst. 1990;82(22):1765-1769.
5 Prescher G et al. Lancet. 1996;347(9010):1222-1225.
6 Worley LA et al. Clin Cancer Res. 2007;13(5):1466-1471.
7 Onken MD et al. Cancer Res. 2004;64(20):7205-7209.
8 Petrausch U et al. Eye (Lond). 2008;22(8):997-1007.
9 Onken MD et al. Ophthalmology. 2012;119(8):1596-1603.
10 Schopper V, Correa ZM. Int J Retin Vitr. (2016) 2:4. doi: 10.1186/s40942-016-0030-2.
11 Field MG et al. Clin Cancer Res. 2016;22(5):1234-1242.
12 Field MG et al. Oncotarget. 2016;7(37):59209-59219.
13 Gezgin G et al. JAMA Ophthalmol. 2017;135(6):541-549.
14 Moschos MM et al. Anticancer Res. 2018;38(7):3817-3824.
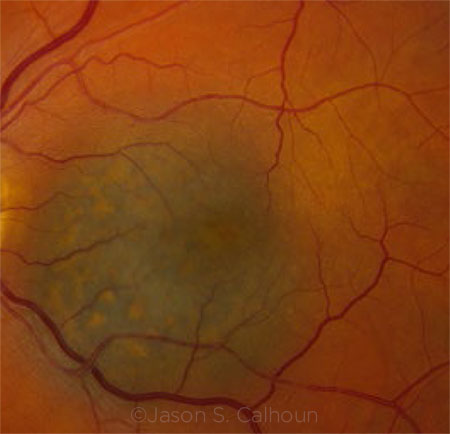 |
UVEAL MELANOMA. Prognostic testing for uveal melanoma may help bring personalized medicine to patients.
|
DR. ROOZBAHANI AND DR. RANDLEMAN ON REFRACTIVE SURGERY AND CORNEA
Corneal Biomechanics for Ectasia
Advances in direct corneal biomechanical measurements have the potential to change both the paradigms for diagnosis and treatment of ectatic disorders, such as keratoconus, and for ectasia screening for refractive surgery. These conditions are tightly intertwined, and the demand for these services is growing.
The prevalence of myopia is expected to double and affect up to 50% of the U.S. and other populations worldwide by 2050,1 and keratoconus—the leading cause of full-thickness corneal transplantation in the United States—is up to 10 times more prevalent than the previously reported figure of 1 in 2000.2 Thus, in the coming years, more patients will be presenting for refractive surgical correction and will require optimal screening for surgical candidacy. Meanwhile more keratoconus patients can benefit from corneal cross-linking (CXL), especially if they are identified at the earliest stages of disease, when they can get the greatest benefit from the procedure. Corneal imaging, and ultimately corneal biomechanics, lie at the heart of optimizing care for both patient groups.
Biomechanical failure is widely recognized to be the root cause of keratoconus,3 and it is the leading concern for refractive surgery screening for ectasia risk.4 Unfortunately, the refractive surgery screening and keratoconus testing available today measure later-onset morphologic surrogates, such as corneal curvature and thickness, rather than making primary, direct biomechanical measurements of the cornea. Because of this, many otherwise good candidates are refused surgery because they have an atypical corneal curvature, when in fact they would have done well with surgery. But this is changing.
Current in vivo Measurement of Corneal Biomechanical Properties
The ocular response analyzer (ORA, Reichert Technologies), which has been available for many years, is a dynamic bidirectional applanation device that quantifies corneal deformation after air puff–stressing. It measures 2 primary biomechanical variables, corneal hysteresis and corneal resistance factor. These variables have been thoroughly studied, and while they perform well at distinguishing between keratoconic and normal populations, they perform poorly at detection of early ectatic disease. Custom variables derived from comprehensive signal analysis have performed better,5 but further work is necessary to determine the ultimate utility of ORA for screening.
More recently, air puffs have also been combined with Scheimpflug imaging (CorvisST, Oculus Optikgeräte) to evaluate corneal response to perturbation.6 The CorvisST couples with the Scheimpflug camera to provide cross-sectional corneal images during the indentation cycle. This provides a host of parameters that can be evaluated. To date, results are mixed in terms of screening utility for subclinical keratoconus, with some authors finding best results by combining data from the CorvisST with various Scheimpflug parameters.7
What Does the Future Hold?
A variety of different technologies are being explored to assess their utility in directly measuring corneal biomechanics. These include optical coherence tomography–based elastography,8 ultrasound methods,9 and Brillouin microscopy.10-12
Scarcelli and Yun developed Brillouin microscopy as an all-optical, nonperturbative mechanical process that provides high-resolution 3-D corneal mapping. In preliminary analyses, Brillouin microscopy has been able to differentiate normal and keratoconic corneas in vivo and has demonstrated the focal nature of keratoconus, with the conical region demonstrating significant reduction in corneal stiffness, while the nonconical regions appeared similar to normal control corneas.11 Brillouin microscopy has also proved to be sensitive to identifying the stiffening effects of CXL ex vivo.12
Conclusion
Direct and accurate corneal biomechanical measurements could create a paradigm shift in the way corneas are evaluated in a large variety of clinical settings. Such measurements may aid in early identification of corneal ectasia, thereby improving the management of keratoconus and making screening for refractive surgery even more precise. Moreover, the process of correlating biomechanical profiles and morphological behavior of the cornea, combined with the development of a predictive modeling based on this connection, could ultimately lead to completely individualized refractive procedures and patient-specific CXL treatment protocols.13
___________________________
1 Holden BA et al. Ophthalmology. 2016;123(5):1036-1042.
2 Godefrooij D et al. Am J Ophthalmol. 2017;175:169-172.
3 Scarcelli G et al. Invest Ophthalmol Vis Sci. 2014;55(7):4490-4495.
4 Randleman JB et al. Ophthalmology. 2008;115(1):37-50.
5 Dupps WJ Jr., Seven I. Trans Am Ophthalmol Soc. 2016;114:T1-1-T1-16.
6 Valbon BF et al. J Refract Surg. 2014;30(7):468-473.
7 Vinciguerra R et al. J Refract Surg. 2017;33(6):399-407.
8 Ford MR et al. J Biomedical Optics. 2011;16(1):016005.
9 He X, Liu J. Invest Ophthalmol Vis Sci. 2009;50(11):5148-5154.
10 Scarcelli G, Yun SH. Nat Photonics. 2007;2:39-43.
11 Scarcelli G et al. JAMA Ophthalmol. 2015;133(4):480-482.
12 Scarcelli G et al. Invest Ophthalmol Vis Sci. 2013;54(2):1418-1425.
13 Seven I et al. Invest Ophthalmol Vis Sci. 2016;57(14):6287-6297.
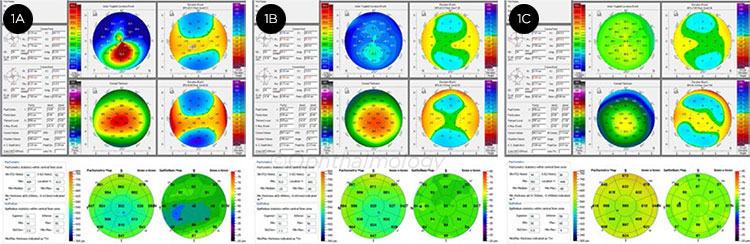 |
KERATOCONUS. Representative images from patients with highly asymmetric keratoconus (1A: affected eye, 1B: clinically unaffected eye) and a normal control eye (1C). While keratoconus can be identified using a variety of devices, identifying eyes at the earliest stages of disease remains challenging, even when using multiple technologies.
|
Meet the Experts
Sanjay G. Asrani, MD Professor of ophthalmology and head of the Glaucoma OCT Reading Center, Duke University at the Duke Eye Center in Durham, N.C. He is also medical director of the Duke Eye Center of Cary, N.C. Relevant financial disclosures: Aerie: C; Bausch + Lomb: C; Camras Vision: C; Noveome Biotherapeutics: C; Regenexbio: C.
Zélia M. Corrêa, MD, PhD The Tom Clancy Professor of Ophthalmology at the Wilmer Eye Institute in Baltimore. Relevant financial disclosures: Castle Biosciences: C.
Mary Lou Jackson, MD Clinical associate professor of ophthalmology and visual sciences at the University of British Columbia, Vancouver. Relevant financial disclosures: None.
Lylas G. Mogk, MD At the Center for Vision Rehabilitation and Research at Henry Ford Health System in the Greater Detroit area. Relevant financial disclosures: None.
J. Bradley Randleman, MD Professor of ophthalmology; director, cornea, external disease, and refractive surgery; and medical director of the University of Southern California (USC) Roski Eye Beverly Hills Clinic. Relevant financial disclosures: None.
Mehdi Roozbahani, MD Cornea fellow at USC Roski Eye Institute in Los Angeles. Relevant financial disclosures: None.
Full Financial Disclosures
Dr. Asrani Aerie: C; Bausch + Lomb: C; Camras Vision: C; Noveome Biotherapeutics: C; Regenexbio: C.
Dr. Corrêa Castle Biosciences: C.
Dr. Jackson Astellas: C.
Dr. Mogk None.
Dr. Randleman None.
Dr. Roozbahani None.
Disclosure Category
|
Code
|
Description
|
Consultant/Advisor |
C |
Consultant fee, paid advisory boards, or fees for attending a meeting. |
Employee |
E |
Employed by a commercial company. |
Speakers bureau |
L |
Lecture fees or honoraria, travel fees or reimbursements when speaking at the invitation of a commercial company. |
Equity owner |
O |
Equity ownership/stock options in publicly or privately traded firms, excluding mutual funds. |
Patents/Royalty |
P |
Patents and/or royalties for intellectual property. |
Grant support |
S |
Grant support or other financial support to the investigator from all sources, including research support from government agencies (e.g., NIH), foundations, device manufacturers, and/or pharmaceutical companies. |
|