Download PDF
A look at some successes, continuing challenges, and a powerful new tool that is reshaping the research.
Precision medicine. Personalized medicine. Individualized medicine. Whatever the name, the goal is the same: to bring the entire weight of knowledge about molecular and cell biology, genetics, and genomics to bear, one person at a time, against previously intractable human disorders.
In the 13 years since completion of the $3 billion project to sequence the human genome, researchers have identified hundreds of genes involved in the pathogenesis of diseases. In ophthalmology, these faulty genes are implicated in diseases ranging from rare hereditary syndromes to common conditions such as myopia, primary open-angle glaucoma, and age-related macular degeneration (AMD).
To date, however, these discoveries have not been translated into new modes of personalized ophthalmic care, with a handful of exceptions.
In Search of the Optimal Target
The first-ever randomized controlled phase 3 clinical trial of gene replacement therapy successfully used a recombinant adeno-associated virus (AAV) to deliver a healthy gene to the retinas of children with Leber congenital amaurosis (LCA). The treatment raised levels of a missing protein and restored small amounts of functional vision.1 Spark Therapeutics, the trial’s sponsor, is in the process of securing federal marketing approval for the therapy.
Today’s targets. Single-gene diseases like LCA are better targets for conventional gene therapy than are multigene, multifactorial diseases such as AMD, said Suber S. Huang, MD, MBA, at the Retina Center of Ohio in Cleveland.
“At present, gene therapy is an approach best used for diseases caused by single-gene defects,” Dr. Huang said. “If only one thing is broken, however difficult it is to fix, that’s still only one thing that needs fixing. Repair, replace, or substitute for the broken part, and the car will work—theoretically, as good as new.”
Clinical trials in the works. A number of single-gene trials are under way around the world, including trials for X-linked retinoschisis, achromatopsia, Stargardt disease, Usher syndrome, Leber hereditary optic neuropathy (LHON), choroideremia, and retinitis pigmentosa (RP).2 For instance, Spark Therapeutics is planning trials that will employ the same AAV used in its LCA study to deliver functioning genes to patients with choroideremia and LHON.
In contrast, only 1 ocular gene therapy trial is targeting AMD. In this study, Australian researchers are testing whether a viral vector carrying a gene for a VEGF receptor (sFLT-1) can raise the receptor concentration sufficiently to boost the effectiveness and durability of anti-VEGF injection therapy.2
Tomorrow’s goals. The next wave of innovations in the field is expected to broaden the number of diseases in which gene-based approaches and molecular medicines might be harnessed therapeutically, said Stephen H. Tsang, MD, PhD, at Columbia University. “The technology is emerging quickly, both in the diagnostics and possible interventions. It’s not so far away now,” said Dr. Tsang, one of the pioneers in genome surgery (see “Next-Generation Genomic Editing”).
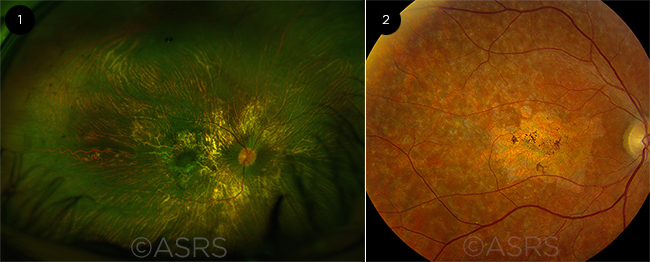 |
SINGLE-GENE TARGETS. Disease such as (1) choroideremia and (2) Stargardt disease pose good targets for conventional gene therapy. Figure 1 was originally published in the ASRS Retina Image Bank. Andrea Elizabeth Arriola-Lopez, MD, MSc. Choroideremia. Retina Image Bank. 2016; Image Number 26396. Figure 2 was originally published in the ASRS Retina Image Bank. Carl C. Awh, MD. Stargardt Disease. Retina Image Bank. 2013; Image Number 5150. © The American Society of Retina Specialists.
|
Oncology: The Future Is Now
The future has already arrived in ocular oncology, as genetic profiling of tumors—and, sometimes, of patients as well—is routinely used to guide treatment and counseling decisions, said Carol L. Shields, MD, at Wills Eye Hospital in Philadelphia.
Success with uveal melanoma. “We’ve been using genomics in the management of uveal melanoma since 2005,” Dr. Shields said. “And since then, we’ve been learning a lot about mutational events that lead to either high risk for metastasis or high risk for other types of cancers associated with uveal melanoma.”
Risk of metastasis. The groundwork for today’s protocols was laid in the late 1990s by European researchers, who found that uveal tumors containing certain gene defects on chromosome 3 were associated with a higher risk of metastasis, Dr. Shields said. Since then, mutated genes on chromosomes 6 and 8 have also been found to increase risk.
“Before treating a uveal melanoma, we sample it and send it either for DNA or RNA testing and get results back that tell us if that melanoma is at risk for spread,” she said. If that’s the case, the patient is placed on a protocol designed to reduce the metastatic risk.
Dr. Shields noted, “I always tell patients that I do genetic testing for 2 reasons. Number one, to identify the high-risk patients and prevent their metastasis if possible. Second, I do it to identify those patients at low risk for metastasis, because knowing that will give them peace of mind. To me, that’s smart medicine.”
Risk of BAP1 cancer predisposition syndrome. More recently, researchers discovered that a genetic variant that was thought to occur spontaneously in uveal tumors might actually be hereditary, she said. The gene governs the activity of a tumor suppressor called BRCA1-associated protein 1 (BAP1).
Studies of families with uveal melanoma have found that people born with a mutant BAP1 gene are at risk not only for uveal melanoma but also for multiple other cancers, including mesothelioma, pancreatic cancer, cutaneous melanoma, and renal cell carcinoma. Dr. Shields and her colleagues at Wills Eye Hospital recently encountered an unusual case in which a woman with a germline BAP1 mutation had 2 independent melanomas in a single eye, suggesting that multiple tumors in the same eye might be a characteristic of the syndrome, she said.
“This is a really fairly new syndrome that was discovered largely by coincidence, when it was noted that multiple family members developed uveal melanoma, and they were also developing other cancers,” Dr. Shields said. “We never knew before that there was any genetic predisposition to uveal melanoma.”
In a study published in 2015, scientists combined genomic sequencing, birth and death records, and genealogical research to track identical copies of a mutant BAP1 gene through several generations of a large, widely scattered U.S. family. They traced the cancer syndrome gene to a couple born in Germany who immigrated to the United States in the 1700s. Through their 10 children, the germline mutation has been passed on to an estimated 80,000 descendants.3
A blood test to rule out this cancer syndrome is necessary if a patient’s uveal tumor contains a BAP1 variant, Dr. Shields said. “If [variant] BAP1 is present in the patient’s blood, we screen the whole family, and anyone who carries the mutation is going to get renal ultrasound, lung evaluation, an eye evaluation to check for the 3 most common malignancies, plus a skin evaluation,” she said.
Tailoring treatment. Laboratory and molecular biology techniques commonly used in genetic research studies also help ocular oncologists individualize treatment, Dr. Shields said.
Mice as therapeutic surrogates. For instance, before beginning local therapy against a uveal melanoma, Dr. Shields sends a tumor sample to a laboratory to determine which chemotherapeutic agents are most effective at killing tumor cells. “The lab grows the melanomas in mice to test which combination of agents is best for that patient’s melanoma.” Thus, she said, “When the patient gets a metastasis, we already know what medications are going to work.”
Genetic and cellular biomarkers. For similar reasons, the lab also studies the tumor cells’ genetic characteristics and their surface biomarkers, Dr. Shields said. Biomarker profiling is particularly helpful with conjunctival melanoma because cancer cells that are positive for the BRAF gene, which has been linked to conjunctival melanoma, are susceptible to vemurafenib, she said.
A note on counseling. Ocular oncologists are well aware of the implications of this new world of personalized medicine. For instance, all children with bilateral retinoblastoma, and about 15% of children with unilateral disease, have germline mutations that led to their tumors, Dr. Shields said. “In addition to sampling the tumors for genetic testing, we always sample their blood, too,” she said. “This is really important. If they carry it in their blood, then they’re at risk to [eventually] pass it on to their kids.”
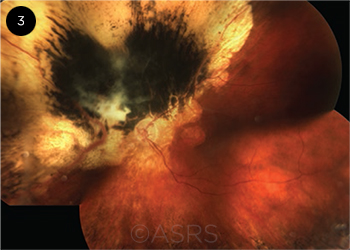 |
CANCER SYNDROME. A patient whose uveal tumor contains the genetic variant known as BAP1 is also at risk of developing multiple other malignancies. This image was originally published in the ASRS Retina Image Bank. Ellen Redenbo, CRA, CDOS, ROUB. Choroidal Melanoma. Retina Image Bank. 2014; Image Number 19261. © The American Society of Retina Specialists.
|
AMD and DR: Still Waiting
But as Dr. Huang noted, the situation is different in other areas of ophthalmology. For instance, in retina, even though the genetics of various retinal diseases are being investigated, genetic testing is not useful at present for managing such multifactorial diseases as AMD and diabetic retinopathy (DR), either for diagnosis, prediction of progression, or prediction of success or harm with various treatments, said Emily Y. Chew, MD, at the National Eye Institute.
“I would love to be able to genotype someone and say, ‘You’re going to have this disease, and it’s going to progress a certain amount,’” Dr. Chew said. “But with these complex diseases, we’re just not able to do that yet.”
Dr. Huang agreed. “For AMD, roughly 36 causative genetic loci have been identified. Each gene variant may be fully or partially expressed in any given individual, and each gene or gene product interacts with the others and with environmental factors that are not yet quantifiable,” he said. “Even if you could say that 12 of the loci were severely affected, and 12 were moderately affected, and 12 were minimally affected, you wouldn’t know what that meant for the development or prognosis of disease for any given individual.”
Clues from gene expression? Even so, Pravin U. Dugel, MD, a retina specialist in Phoenix, expects genetic studies to prove useful down the line for AMD and DR, which he called ophthalmology’s “bread-and-butter diseases.” As he noted, “There’s a great need to understand more about these diseases so we can individualize and personalize the treatment.”
For instance, responses to anti-VEGF injection therapy vary widely, Dr. Dugel said. Studies of gene expression might clarify the reasons for this—and, ideally, they would enable physicians to stage the diseases and customize treatments. “We know that not all AMD eyes behave the same. Phenotypically, they may look the same, and that’s really the problem. We cannot predict how phenotypically indistinguishable eyes will behave.”
Dr. Dugel added, “With diabetic macular edema, perhaps the disease goes from a phase where it’s permeability driven to one that is more inflammation driven. Drug effectiveness may depend on recognizing the proper disease phase. This means choosing the appropriate drug not only for the appropriate patient but also for the appropriate disease phase. This is personalized medicine. Currently, we do not have such granularity and simply lump everything together.”
 |
ELUSIVE. Personalized treatment is not yet available for multifactorial diseases such as diabetic retinopathy (shown here) and AMD. This image was originally published in the ASRS Retina Image Bank. Michael P. Kelly, FOPS. Proliferative Diabetic Retinopathy. Retina Image Bank. 2012; Image Number 774. © The American Society of Retina Specialists.
|
Next-Generation Genomic Editing
Investigators are continuing to work with viruses as delivery and vector systems, as with the research on LCA.
But what if scientists had a straightforward way to directly insert new DNA into the patient’s genome, in the exact spot where it should be? After reports in 20124 and 20135 that this type of in vivo gene editing is indeed possible, other researchers quickly embraced the technique, called CRISPR/Cas9 (for “clustered regularly interspaced short palindromic repeats coupled with the bacterial nuclease Cas9”). A PubMed search on the term CRISPR/Cas9 yields more than 2,000 studies published since 2012.
How CRISPR/Cas9 works. This powerful new tool is a bacteria-derived system that can be designed to recognize any DNA sequences. These designer RNAs act as guides, directing the bacterial nuclease to corresponding locations in the human genome. It has been described as the “simplest genome-editing tool to work with because it relies on RNA-DNA base pairing, rather than the engineering of proteins that bind particular DNA sequences.”6
The Cas9 cleaves both strands of DNA at the targeted site, and this either eliminates a dominant disease-causing gene variant or allows the cell’s endogenous repair mechanisms to insert a new, healthy version there as it mends the break. The cell builds this new gene based on a template inserted into the cells as part of the treatment.7
Call it genome surgery. Thanks to CRISPR/Cas9, the concept of precision medicine has undergone a paradigm shift, Dr. Tsang said. “Until CRISPR came along, you could only do single-gene supplementation. Previously, we were practicing genetic medicine or gene supplementation therapy,” he said. “Now we’re able to do genome surgery.”
The flood of research papers on CRISPR/Cas9 includes reports that it could be used to modify zygotes to create transgenic animals, which in some cases passed the revised genome on to offspring. The prospect of germline editing in humans is controversial, and some countries have banned it.6 (China has not, and a research group from that country has reported that it had applied CRISPR/Cas9-based gene editing in human embryos in an investigation of DNA repair mechanisms.8)
First target: ophthalmology? “The pioneers of genome surgery believe that the first therapeutic use of CRISPR will be to treat an eye disease,” Dr. Tsang said. “Our study [on RP] demonstrated that the initial steps are feasible.”
Retinitis pigmentosa. Dr. Tsang and his colleagues (Vinit B. Mahajan, MD, PhD, and Alex Bassuk, MD, PhD, both at the University of Iowa) used CRISPR/Cas9 gene editing to successfully insert a healthy RPGR (RP GTPase regulator) gene into induced pluripotent stems cells derived from a patient with X-linked RP.9 The goal is a clinical trial in which autologous stem cells would undergo genome surgery and be transplanted into patients to treat their RP, he said.
Corneal dystrophies. A California company that specializes in pre–refractive surgery genetic tests is conducting preclinical studies to validate CRISPR/Cas9-based gene editing to correct Meesmann epithelial corneal dystrophy and other dominantly inherited ocular surface diseases, said Tara Moore, PhD, at Avellino Labs in Menlo Park, Calif.
Dr. Moore and her colleagues at Ulster University in Northern Ireland reported earlier this year that their CRISPR/Cas9-based gene editing technique successfully disrupted a Meesmann-causing gene, KRT12, in mice.10
“A clinical trial is the next step for us, and success would really open up the potential to offer all our patients a much-awaited therapy,” Dr. Moore said. “Now we are in the process of showing that we can use gene editing to remove the mutation from a sufficient number of limbal stem cells to stop the progression of the disease or even to reverse its effects.”
In addition to Meesmann, the group is targeting Fuchs’ endothelial corneal dystrophy and dystrophies associated with transforming growth factor beta mutations, as well as retinal dystrophies, she said.
Other studies. Other genome surgery companies have active research programs aimed at conducting trials of gene editing to correct early-onset retinal dystrophies. For instance, Editas Medicine has said its first trial will be in patients with LCA and could begin this year.
 |
GENOME SURGERY. Researchers have used CRISPR/Cas9 to perform genome surgery in cells derived from a patient with retinitis pigmentosa (shown here). This image was originally published in the ASRS Retinal Image Bank. John W. Kitchens, MD. Autosomal Dominant Retinitis Pigmentosa. Retina Image Bank. 2014; Image Number 18050. © The American Society of Retina Specialists.
|
Additional Thoughts
“Fundamental insights into the nature of gene regulation, aging, and cancer, coupled with technological advances in computational biology, gene editing, and genomic informatics, are driving a pace of discovery that we have never seen before,” said Dr. Huang.
Nonetheless, he and Dr. Chew cautioned that talk about personalizing clinical care based on genetic research and testing remains premature for most areas of ophthalmology. “Clearly, genetics is very important for us to do,” Dr. Chew said. With regard to AMD, she added, “I’m still strongly in support of doing it for research purposes, to understand and learn more about AMD and its different pathways. But to help us guide personalized medicine—we’re not there yet.”
And Dr. Huang said that he is concerned that focusing on personalizing treatments could override our understanding of how advances in ophthalmic research need to be woven together to produce new clinical treatments. “With each new discovery, we find that the eye is even more complex than we thought. A term like personalized medicine is a wonderful, aspirational concept, but it doesn’t reflect how far we have to go before we can incorporate our understanding of the body’s intricate, complex processes into safe, effective, and individualized plans of treatment.”
___________________________
1 Weleber RG et al. Ophthalmology. 2016;123(7):1606-1620.
2 Huang SS et al. Asia Pac J Ophthalmol. 2016;5(4):227-228.
3 Carbone MC et al. PLoS Genet. 2015;11(12):e1005633. doi:10.1371/journal.pgen.1005633.
4 Jinek M et al. Science. 2012;337(6096):816-821.
5 Mali P et al. Nat Methods. 2013;10(10):957-963.
6 Lanphier E et al. Nature. 2015;519(7544):410-411.
7 Chrenek MA et al. Asia Pac J Ophthalmol. 2016;5(4):304-308.
8 Liang P et al. Protein Cell. 2015;6(5):363-372.
9 Bassuk AG et al. Sci Rep. 2016;6:19969. doi:10.1038/srep19969.
10 Courtney DG et al. Gene Ther. 2016;23(1):108-112.
The Precision Medicine Initiative
Precision medicine got a boost in the 2015 State of the Union address, when President Barack Obama announced establishment of a federally driven Precision Medicine Initiative.1
Huge research cohort. As the initiative’s central research element, the National Institutes of Health will recruit an unprecedented cohort of 1 million or more people to participate in longitudinal studies of the ways in which genomics, health behaviors, and lifestyle/environmental risk factors interact to cause diseases. Participants will be asked to contribute their electronic medical records as well as blood samples for genomic analysis and storage in a central “biobank.”2
Large genomic databases such as this can help reveal previously unsuspected genes behind difficult ocular diseases like AMD, thanks to a statistical technique called GWAS (for “genome-wide association studies”), Dr. Tsang said.
And Dr. Huang noted, “If we’re looking at the future of molecular medicine, this eventually will become computational medicine based on examining the genomes of large numbers of people. That information can be used to tease very, very subtle things from the data. This is something we could never do person by person.”
Streamlining FDA rules. In support of the initiative, the FDA has proposed what it calls “flexible and adaptable” regulatory plans to simplify the agency’s process for approving new tests on next-generation sequencing systems. Approval is necessary before certified commercial laboratories can make whole-genome sequencing widely available to physicians in the United States.
Faster, cheaper. Next-generation sequencers have what’s known as a “massively parallel” design and can sequence thousands of genes simultaneously, and they can do so faster and less expensively than earlier devices. In addition, the reagent cost to sequence an individual’s genome is about $1,500, compared to the $3 billion it cost to do this during the Human Genome Project, Dr. Tsang pointed out.
The FDA’s existing regulations allow exome sequencing on these devices but restrict whole-genome sequencing in the United States to research only, said Dr. Tsang. “The National Health Service in the United Kingdom is doing better than us at this. They have whole-genome sequencing freely available for patients.” He added, “I often tell my patients that if George Washington had lost the war, we would have whole-genome sequencing as a standard of care [in this country].”
___________________________
1 The Precision Medicine Initiative. Accessed Oct. 31, 2016.
2 Precision Medicine Initiative Cohort Program. Accessed Oct. 31, 2016.
|
Meet the Experts
Emily Y. Chew, MD Deputy Director of the Division of Epidemiology and Clinical Applications at the National Eye Institute and chair of the AREDS2 studies of AMD interventions. Relevant financial disclosures: None.
Pravin U. Dugel, MD Managing partner at Retinal Consultants of Arizona in Phoenix; physician executive director of the Phoenix Eye Institute at the Banner University Medical Center in Phoenix; and clinical professor at the University of Southern California’s Roski Eye Institute in Los Angeles. Relevant financial disclosures: None.
Suber S. Huang, MD, MBA CEO of the Retina Center of Ohio in Cleveland and chair of the NEI’s National Eye Health Education Program. Relevant financial disclosures: AECOM: C; Biofirst: C; i2i Innovative Ideas: O; Lumoptik: O; Regenerative Patch Technologies: C; RegenixBio: C; Second Sight: C; Spark: C.
Tara Moore, PhD Professor of personalized medicine and director of the Biomedical Science Research Institute at Ulster University in Belfast, Northern Ireland, and director of research and development for Avellino Labs in Menlo Park, Calif. Relevant financial disclosures: Avellino Labs USA: E.
Carol L. Shields, MD Codirector of the Oncology Service at Wills Eye Hospital and professor of ophthalmology at Thomas Jefferson University in Philadelphia. Relevant financial disclosures: Aura Bioscience: C.
Stephen H. Tsang, MD, PhD Attending physician in the Electrodiagnostic Clinic at New York-Presbyterian Hospital and associate professor of ophthalmology and of pathology and cell biology at Columbia University in New York, N.Y. Relevant financial disclosures: None.
For full disclosures and the disclosure key, see below.

|