Introduction
Advances in neuroradiologic techniques have aided in the evaluation of neuro-ophthalmologic conditions, in particular the use of computed tomography (CT) and magnetic resonance imaging (MRI). Effective use of these tools can aid in narrowing differential diagnoses, help to exclude life-threatening conditions, and help to follow treatment response for these conditions. However, requesting the incorrect study may provide incomplete and even misleading information. This overview explains cross-sectional imaging techniques available for the brain and orbits to aid in patient-specific and disease-specific diagnosis.
Computed Tomography
Computed tomography (CT) is a cross-sectional imaging technique that uses X-ray beams and powerful computers to construct images of the body. CT can be acquired rapidly, is typically available 24 hours a day at most hospitals, and is the mainstay of emergent evaluation of the brain and orbits. Its use is particularly helpful in the setting of traumatic and infectious conditions. CT of the head is excellent at detecting acute intracranial hemorrhage, which typically has a high-density appearance. CT is also excellent at detecting fractures.
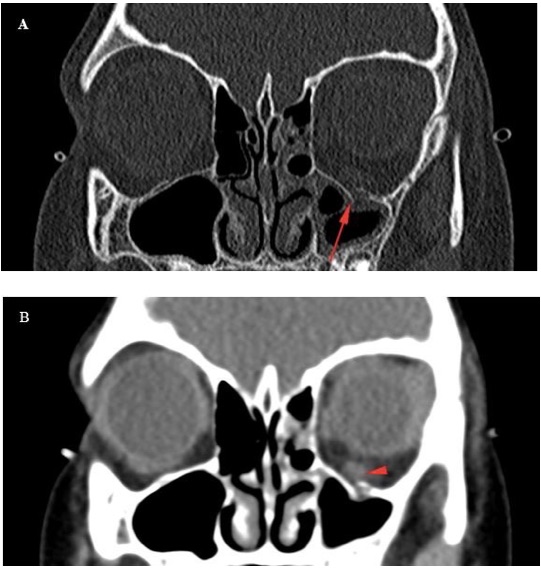
Figure 1. (A) Coronal bone algorithm images of the orbits in a child after trauma shows a discontinuity in the floor of the left orbit. (B) Coronal soft tissue algorithm image of the same location shows asymmetric enlargement of the left inferior rectus muscle. These two images are from the same CT acquisition; however, they are processed differently to accentuate different features.
CT Density and Windows
CT data represents the density of the tissues being evaluated, which is indicated by a density value known as Hounsfield units (HU). Water is defined as 0 HU, and air is defined as -1000 HU. Fat typically has a density of approximately -100 HU, and bone has a density higher than 100 HU. An acute blood clot can have a density of approximately 60 HU (because blood is thicker than water). This is relevant because the vitreous of the eye should be very similar to water; however, a hyphema will have a higher density.
In order to display images where the HU density range can be differentiated by the eye of the reader, it is possible to adjust the imaging appearance with an “imaging window.” Common windows include those that are optimized for soft tissues, for bone, for brain parenchyma (where the window tries to accentuate subtle differences in gray and white matter), and for lungs. While the ophthalmologist does not typically have an interest in the lungs themselves, the lung-window images can be used to detect air in the orbit after trauma. This windowing can be performed through most picture archiving and communication system (PACS) software.
Iodinated Contrast
Iodinated contrast has a high density, and after intravenous injection there will be “enhancement” of the peripheral rim of an abscess, but no central enhancement within the abscess. This is in contradistinction to a phlegmon, which will be an amorphous area of enhancement without discrete drainable fluid collection. Performing a CT of the orbits with and without contrast is typically not needed, because it provides very little additional information and it doubles the radiation dose to the eyes. Performing a contrast-enhanced CT of the head is also rarely indicated, even when there is concern for infection, since a CT without contrast can provide most of the information needed to make a clinical treatment decision. If there is further concern, an MRI with and without contrast is preferred.

Figure 2. Axial CT image of the orbits after contrast administration in a 2-year-old child with eye swelling shows a thin enhancing rim in the medial aspect of the right orbit (red arrow), with a hypoenhancing area between this rim and the lamina papyracea (red arrowhead), representing a subperiosteal abscess originating from ethmoid sinus mucosal disease. Note that there is proptosis, and there is also periorbital/preseptal soft tissue swelling (white arrowhead).
Performing a CT scan with thin-section images immediately after injecting iodinated contrast results in a CT angiogram (see angiography section, Figure 11).
Administration of iodinated contrast can impair renal function, so it is typically avoided in patients with renal failure and must be used with caution in the elderly. If a patient with renal dysfunction has a condition that may require a contrast-enhanced CT, the risk-benefit consideration of administering contrast versus performing a non-contrast study must be evaluated, typically through consultation with the radiologist and possibly with a nephrologist. Patients with a history of anaphylactic reaction to iodinated contrast administration have a contraindication to it; if contrast is needed, pre-treatment protocols exist to decrease the risk of repeat reaction, but cannot entirely prevent a new reaction.
Radiation Safety
CT uses ionizing radiation, which is known to have carcinogenic potential. The risks are relatively small for the individual patient; however, if studies are performed on a large number of patients, the chances increase for radiation-induced complications. Beyond carcinogenesis, radiation to the lens of the eye increases the risk of cataract development. The information provided by appropriately indicated studies outweighs the risks of the radiation, and several important steps can be taken to minimize the overall risk to the population. First, the ophthalmologist should consider whether a study must be performed. If so, there may be a radiation-free alternative (such as ultrasonography or MRI). Second, the radiation dose settings must be patient-specific. For example, a 2-year-old child receiving a CT requires much lower radiation settings than an adult. Third, multi-phase imaging (such as imaging prior to and after contrast administration) should be avoided unless absolutely needed. Awareness of these concepts has been increased by the Image Gently campaign.1,2
Various CT Protocols to Order for Specific Conditions
Several types of CT scans can be performed. The differences include whether or not iodinated contrast is used, the field of view, and the slice thickness. The main field-of-view question relevant to ophthalmology is whether imaging of the brain is required, or imaging of the orbits. Iodinated contrast is commonly used to look for signs of infection/inflammation, and can also be used for vascular opacification in angiographic studies. Table 1 summarizes the main variants of CT scans used in ophthalmologic evaluation. It is important to recognize that due to the radiation dose, dual-phase imaging of the orbits (imaging before and after contrast) is almost never performed with CT scanning, whereas it is common with MRI.
Table 1. Main Variants of Computed Tomography Scans Used in Neuro-Ophthalmologic Evaluation
|
Imaging Study
|
Comment
|
Pro
|
Con
|
CT of the head without contrast
|
Mainstay of evaluating acute neurologic deficits and head trauma
|
Very good bone detail, quick, widely available
|
Radiation, decreased soft tissue detail as compared to MRI, the bones of the skull base can obscure full evaluation of the brainstem and cerebellum.
|
CT of the orbits without contrast
|
Mainstay of evaluating orbital trauma
|
Very good bone detail, quick, widely available
|
Radiation, decreased soft tissue detail as compared to MRI
|
CT of the orbits with contrast
|
Mainstay of evaluating orbital infections
|
Good bone detail, quick, widely available, good at detecting an abscess (and localizing the infectious process between the pre-septal and post-septal space)
|
Radiation, requires intravenous access
|
CT angiogram of the head
|
Mainstay of acute evaluation of vascular abnormalities
|
Quick, widely available
|
Radiation, requires intravenous access
|
CT, computed tomography; MRI, magnetic resonance imaging
Contraindications
There are generally few absolute contraindications to CT scanning. As mentioned, patients with renal dysfunction or history of prior anaphylactic reaction have a strong relative contraindication to iodinated contrast administration. Radiation dose is always a consideration but rarely an absolute contraindication. Patients with DNA repair abnormalities, such as ataxia telangiectasia, should not have CT scans because of their increased risk of carcinogenesis. Patients with germ-line mutations in the retinoblastoma gene are also at increased risk of neoplastic induction by ionizing radiation.
Magnetic Resonance Imaging
MRI is a cross-sectional imaging technique that uses powerful magnets to align the protons in the water molecules into a uniform direction. Short bursts of radio waves are then directed at the target tissues, knocking the protons out of alignment. When the radio waves cease, the protons return to their original magnetized alignment. The changes created by alignment produce a signal that is detected by the scanner. MRI scans typically have multiple different scan alignments that can be used with different MRI sequences and imaging planes, as discussed below. MRI scans are not typically as readily available as CT scans, and may require 30 to 60 minutes of scan time. For this reason, young children often require sedation for scans. MRI far surpasses CT in its ability to characterize soft tissue findings. MRI typically is not as good as CT for imaging fractures and bony detail; however, bone marrow abnormalities can sometimes be better characterized on MRI.
Multiple MRI sequences exist, with the key sequences (T1, T2, etc.) summarized in the following section. It is important to note that each sequence has numerous variations. For instance, there are image acquisition techniques such as spin-echo and gradient echo, and images can be acquired one at a time or in a volumetric manner, among other variations. MR images of the orbit may be accentuated through the use of a surface coil, improving detail. Each of these variations has strengths and weaknesses, and determining the optimal imaging is a multidisciplinary approach that may vary by institution, as well as by disease process.
MRI Sequences: T1
T1 weighted imaging is one of the fundamental MRI imaging sequences. Intracranially, it provides evaluation of gray-white differentiation in the brain, with gray matter relatively darker on T1 images than white matter. Water is typically dark on T1 images. Fat, proteinaceous material, melanin, and gadolinium are among the substances that are bright on T1 imaging. Post-contrast T1 imaging can be used to identify tumors, infection, and inflammation. Identifying post-contrast enhancement can be difficult in a background of fat, such as in orbital cellulitis, due to the bright signal of both the fat and the gadolinium. A “fat-suppressed” T1 image is a sequence where the signal from fat is nullified, and therefore enhancement within the otherwise bright background can be identified.
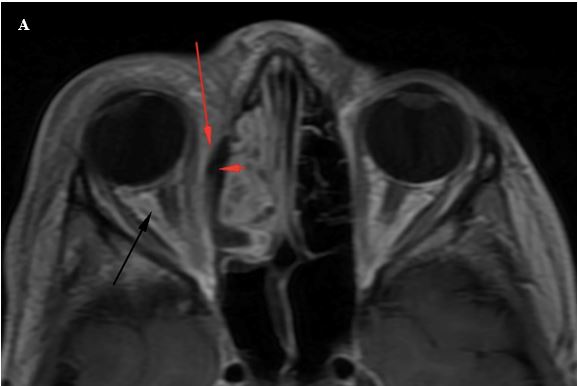
Figure 3A. Axial T1W post-contrast image shows an area of hypointense signal in the medial extraconal fat of the right orbit (red arrowhead). Superficial to this is high-intensity signal in the medial extraconal space (red arrow) and in the intraconal space (black arrow).
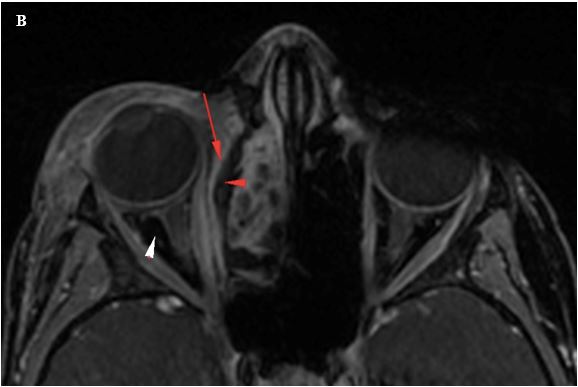
Figure 3B. Axial T1W post contrast image with fat suppression again shows the hypoenhancing fluid collection along the right lamina papyracea, representing a subperiosteal abscess arising from right ethmoid sinus mucosal disease. There is an enhancing rim to this collection (red arrowhead). The retrobulbar fat signal has been suppressed, and is no longer hyperintense (white arrowhead), differentiating areas of hyperintense signal due to post-contrast enhancement, as compared to the intrinsic hyperintense signal of fat.
MRI Sequences: T2
T2-weighted imaging is another of the fundamental MRI imaging sequences. White matter appears darker on T2 images than gray matter. Water, vitreous, and fat are bright on T2 imaging, and this sequence is useful when looking for edema. Edema can be difficult to detect in fat, and fat-suppressed techniques can also be useful when looking for edema to create enhancement from the typically bright signals.
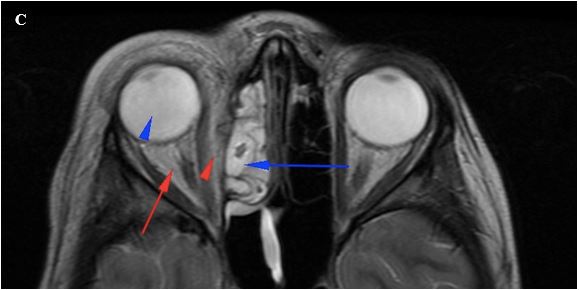
Figure 3C. Axial T2W image shows hyperintense signal within the vitreous (blue arrowhead), and the right ethmoid sinus mucosal disease (blue arrow). Hyperintense signal is noted in the medial extraconal space (red arrowhead) and in the retrobulbar/intraconal soft tissues (red arrow).
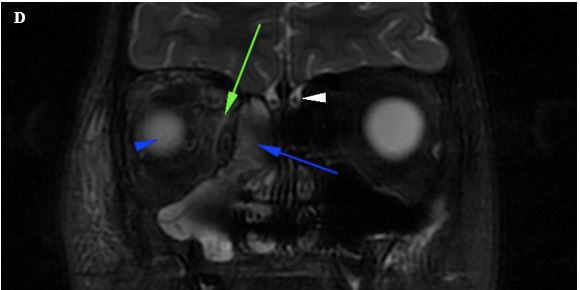
Figure 3D. Coronal T2W image with fat suppression shows the right ethmoid sinus mucosal disease (blue arrow) and hyperintense vitreous (blue arrowhead), similar to the T2W images without fat suppression. As the hyperintense signal of the intraconal fat has been suppressed, the hyperintense signal from edema can be identified (green arrow) in the background of suppressed fat. This image provides a good view of the olfactory nerves (white arrowhead).
MRI Sequences: Fluid Attenuation Inversion Recovery
Fluid attenuation inversion recovery (FLAIR), is an MRI sequence where pulses are used to nullify the signals from fluids such as cerebrospinal fluid (CSF) and water. This is typically done as a variant of a T2 image, where water and CSF appear bright to reduce the brightness of the fluid signal and enhance contrast with the targeted tissue. This sequence is very good at detecting edema in the brain and/or optic nerves, and is an improvement upon regular T2 images. FLAIR sequences are commonly used for the evaluation of brain parenchyma in demyelinating conditions such as multiple sclerosis, whereas T2 images are often better at identifying plaques in the brainstem and cerebellum.
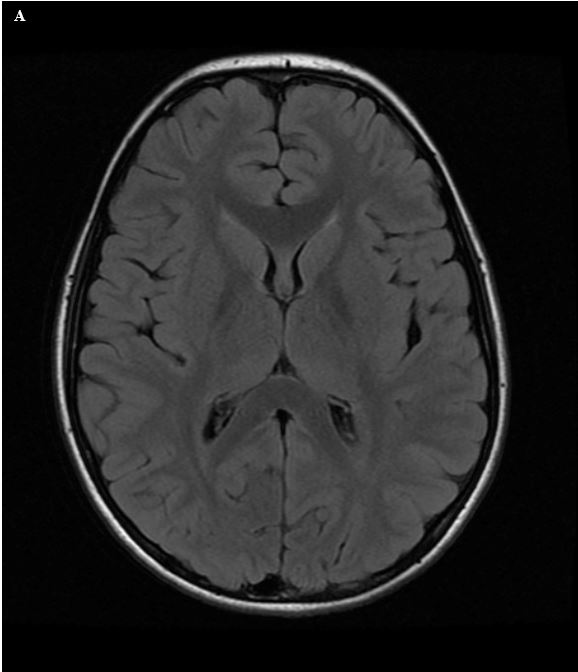

Figure 4. (A) Axial T2W image of the brain shows high-intensity signal from CSF in the ventricles and overlying sulci. (B) FLAIR image shows suppression of the hyperintense signal from CSF. Note that other causes of hyperintense T2 signal, such as the subcutaneous fat, remain bright on the FLAIR images.
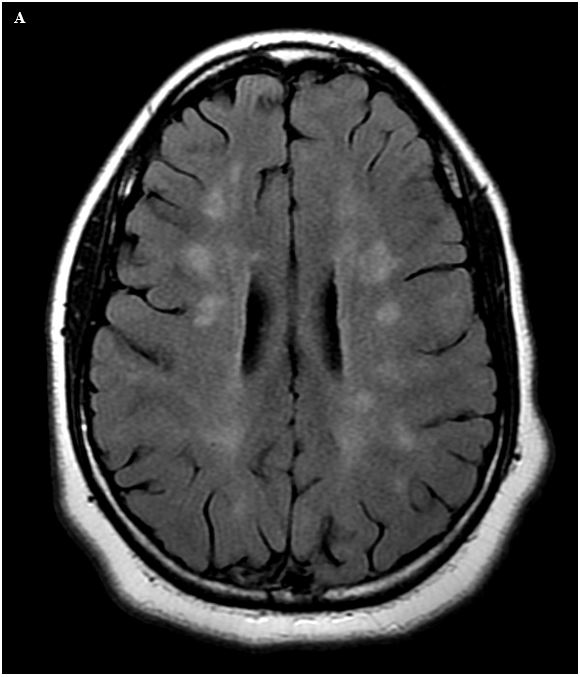
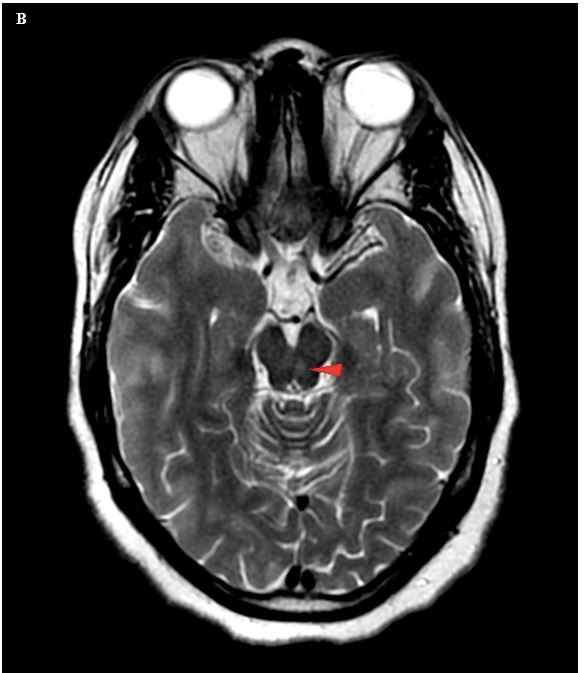
Figure 5. (A) Axial FLAIR image in a 16-year-old female with known multiple sclerosis shows multifocal areas of hyperintense signal in the deep white matter of both cerebral hemispheres. (B) Axial T2W image shows a hyperintense focus in the mesencephalon, slightly to the left of midline, which was not present on the patient’s prior MRI, representing a new demyelinating plaque. Given the proximity to the expected location of the oculomotor nucleus, this likely accounts for the patient’s new diplopia. This finding was not well seen on FLAIR images, and it is important to remember that T2W images often perform better than FLAIR in detecting MS plaques in the brainstem and cerebellum.
MRI Sequences: Diffusion Weighted Imaging
Diffusion weighted imaging (DWI) is a technique that quantifies the Brownian motion of water. Brownian motion is the random motion of particles when suspended in a fluid that results from the particles’ collision with the other molecules in the liquid. The most well-known use of this technique is in identifying acute infarction (stroke). This technique also can be used in the evaluation of the purulent core of an abscess, to characterize an epidermoid cyst, and to characterize tumor cellularity. Apparent diffusion coefficient (ADC) is a sequence processed from DWI to provide quantified information about the degree of Brownian motion, which has been used to characterize tumor cellularity.
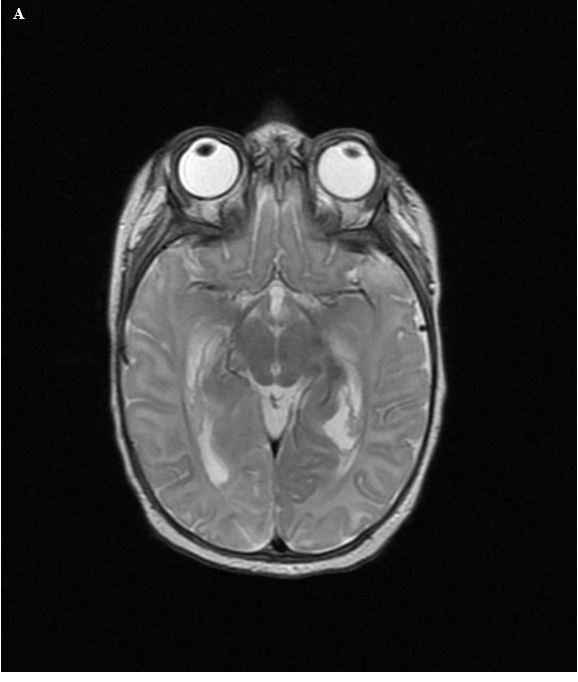

Figure 6. (A) Axial T2W image in a 3-month-old child with eye movement abnormalities in the setting of meningitis shows no definite abnormalities. (B) Axial diffusion-weighted imaging shows abnormalities in the right occipital lobe, representing a stroke likely due to vasospasm in the right posterior cerebral artery. Upon close inspection, the original T2W image (Fig. A) had a slight asymmetry; however, it was much more subtle than the diffusion abnormality.
MRI Sequences: Cranial Nerve Imaging (CISS, FIESTA)
High-resolution imaging can be performed to evaluate cranial nerves and other small structures. A variety of approaches exist; however, currently the most common set of sequences are variations of balanced steady-state free precession techniques, which are known by vendor acronyms such as FIESTA (on GE scanners) and CISS (on Siemens scanners). These provide excellent detail about the cisternal segments of cranial nerves and, with refined technique, can evaluate the interdural and extradural segments of the cranial nerves.3,4
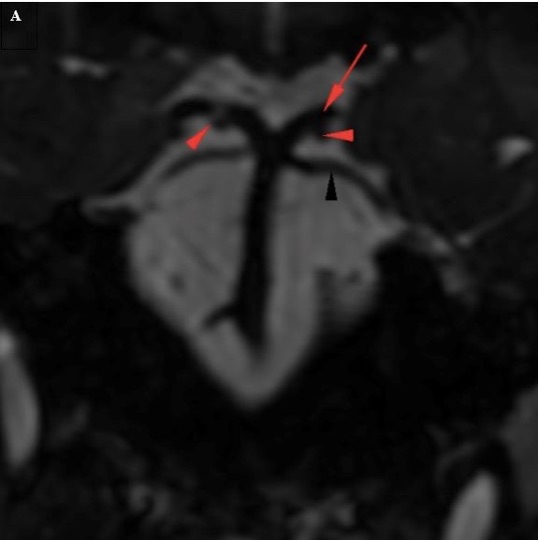
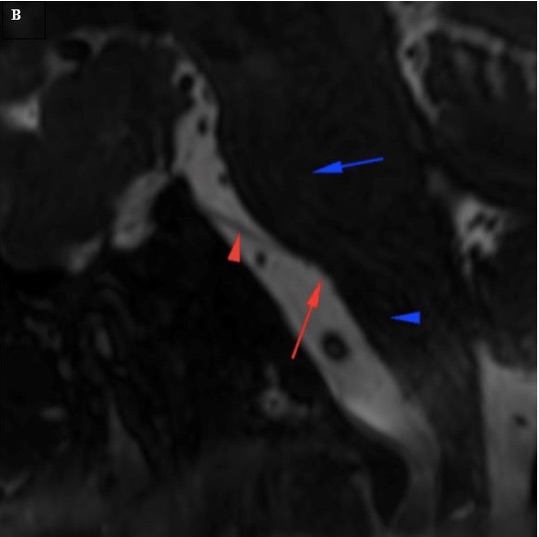
Figure 7. (A) Coronal cranial nerve imaging shows the oculomotor nerves (red arrowheads) coursing between the posterior cerebral arteries (red arrow) and superior cerebellar arteries (black arrowhead). (B) Sagittal oblique cranial nerve imaging shows the cisternal segment of the abducens nerve (red arrow). The nerve arises from the pontomedullary sulcus (red arrow), at the junction of the pons (blue arrow) and the medulla oblongata (blue arrowhead).
MRI Sequences: Susceptibility Weighted Imaging
Susceptibility weighted imaging (SWI) is a technique that is very sensitive for identifying areas of hemosiderin deposition and dystrophic mineralization within the brain. This can be useful in the setting of traumatic brain injury, neurocutaneous disorders that result in dystrophic mineralization (such as tuberous sclerosis complex and Sturge-Weber syndrome), and in evaluation of intracranial neoplasms. If SWI is not available, a similar but less-sensitive sequence known as gradient-recalled echo (GRE) or T2-star imaging can be used.
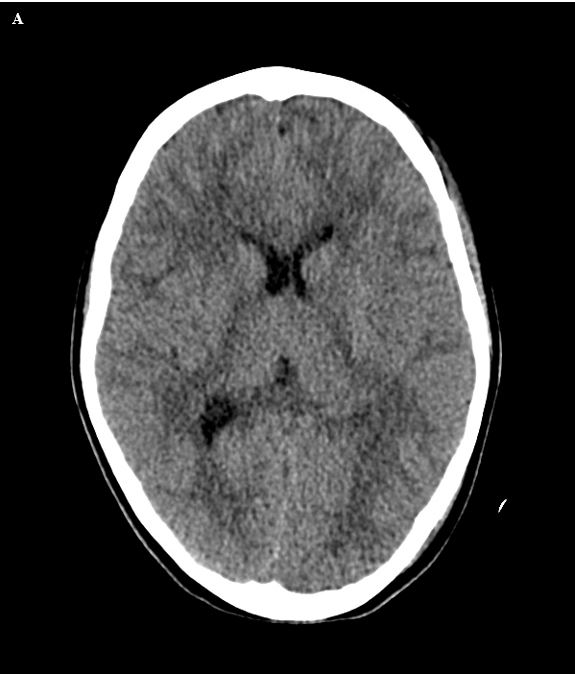
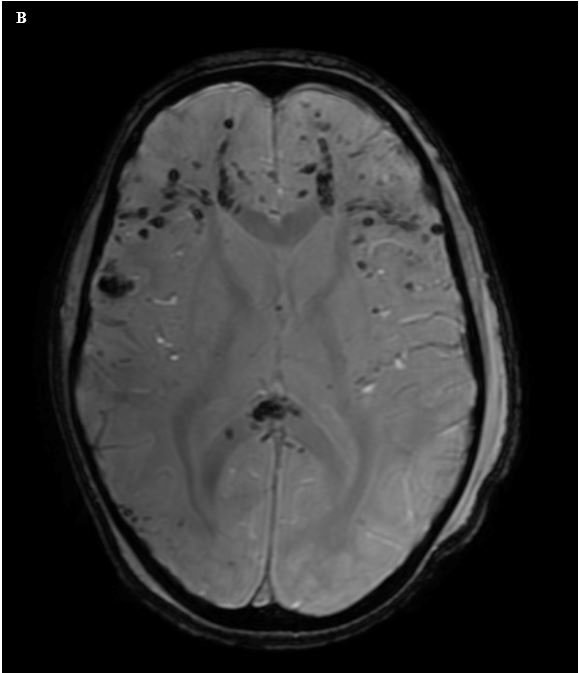
Figure 8. (A) Axial CT image in an 8-year-old patient with neurologic deficits after motor vehicle accident shows no clear evidence of acute intracranial hemorrhage. (B) Axial susceptibility weighted image shows numerous foci of hypointense signal in the bilateral frontal white matter, and in the splenium of the corpus callosum, representing petechial hemorrhages in the setting of diffuse axonal injury.
Advanced MRI Techniques: Diffusion Tensor Imaging
Diffusion tensor imaging (DTI) is an advanced MRI technique that is a complex variant of DWI. DTI quantifies water movement in different directions. If water can move equally in all directions, it is known as isotropic movement. Within white matter, however, water moves more freely along the long axis of the axon rather than perpendicular to the axon. This anisotropic water movement can be used to detect white matter fiber bundles such as the corpus callosum and optic radiations. Uses for DTI include determining if white matter has developed normally, and if specific white matter tracts are impacted by specific pathologic conditions.5
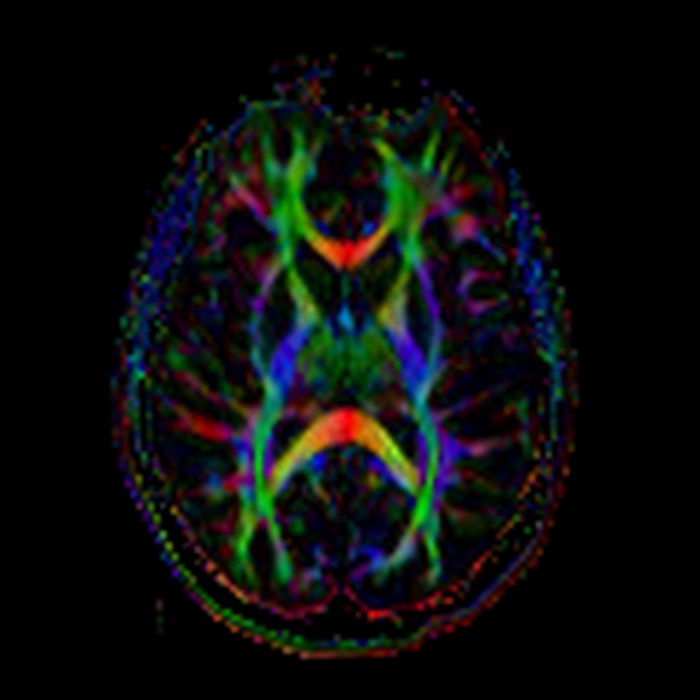
Figure 9. Axial directionally encoded fractional anisotropy map from a diffusion tensor imaging study shows white matter tracts based upon the predominant orientation of the fibers. Red represents transversely oriented fibers, such as in the genu and splenium of the corpus callosum. Blue represents cranio-caudally directed fibers, such as the descending fibers of the corticospinal tracts within the posterior limb of the internal capsules. Green represents anterior-posteriorly directed fibers, including the optic radiations extending posteriorly from the lateral geniculate nucleus to the juxtacalcarine occipital cortex.
Advanced MRI Techniques: Functional MRI
Functional MRI (fMRI) is an advanced technique that attempts to identify brain locations that carry out specific functions. It makes use of the blood oxygen level dependent contrast (BOLD). The hemoglobin that carries oxygen contains iron, and the magnetic signal of iron is different when carrying oxygen than when it is oxygen free. This difference can be detected by the scanner. Since oxygen utilization varies within the different sections of the brain, fMRI allows for a differentiation between tissues based upon activity. Currently, this is primarily used in research applications, and it has been useful in demonstrating which areas of the brain are used during specific physical and mental tasks. For instance, comparing images of children with learning disabilities to those of children without those disabilities has allowed better understanding of the complex nature of learning to read. This technique can also be used to identify visual cortex. Once the areas of cortical activation are identified by fMRI, DTI can be used to find the white matter fibers that arise from this cortex. This can be challenging to perform on children, but it has been successful in certain situations. This technique holds promise for future applications and is under continuous study; however, at the present time, visual fMRI is mostly a research tool that is rarely used clinically.6,7

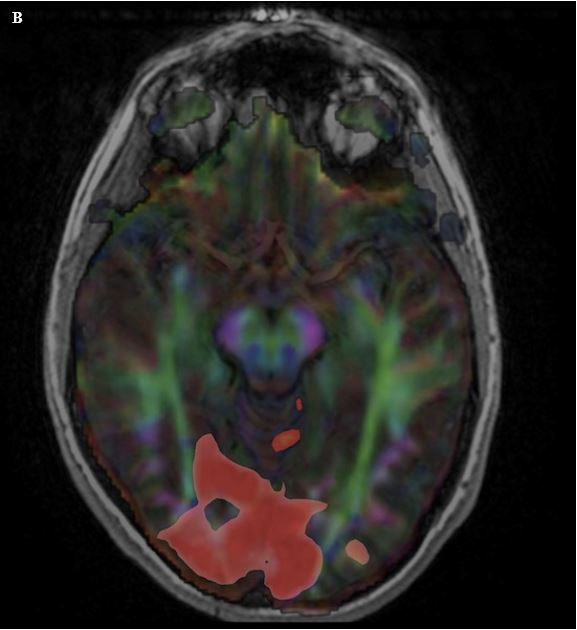
Figure 10. (A) Sagittal T1W image of the brain with overlay of data from functional mapping of the visual cortex (orange overlay). This technique can be used to identify the areas of brain involved with a specific function. (B) Axial T1W image with visual cortical fMRI overlay (red overlay) and also with overlay of diffusion tensor imaging (multi-color overlay). By combining the fMRI data with DTI, it is possible to then identify the white matter tracts that extend to these areas of cortical activation.
Intravenous Contrast
Intravenous contrast can be used in MRI to identify areas of inflammation; however, instead of iodinated contrast in CT, MRI uses gadolinium chelates. Gadolinium chelates do not cross the blood-brain barrier, so areas of inflammation where there is loss of the blood-brain barrier, such as an active demyelinating plaque in multiple sclerosis, will show post-contrast enhancement. Many neoplasms without an intact blood-brain barrier will enhance. In adult brain tumors, typically only high-grade tumors enhance; however, many low-grade pediatric brain tumors (such as pilocytic astrocytoma) will have post-contrast enhancement. Unlike CT, where imaging before and after contrast is rarely performed as it increases the overall dose of radiation, contrast-enhanced MRI studies nearly always have pre-contrast imaging.
Renal failure is a contraindication to gadolinium administration, but for different reasons than for iodinated contrast used in CT scans. Gadolinium is excreted by the kidneys, and with impaired renal function there is delayed clearance. With this prolonged circulation time, gadolinium can unchelate. Free (unchelated) gadolinium can diffuse across capillary membranes into the interstitial space and result in a fibroblastic inflammatory response. This can lead to a condition known as nephrogenic systemic fibrosis (NSF).
It is also important to know that in the United States, most intravenous gadolinium agents have not been formally labeled for use in children under 2 years of age. While not formally labeled for this indication, gadolinium is commonly used in children younger than 2 in whom there is concern for infectious, inflammatory, or neoplastic processes.
Various MRI Protocols for Different Conditions
MRI of the Brain
- Can be performed without contrast, or with and without contrast. Contrast is typically administered when looking for signs of infection, a noninfectious inflammatory process, or a neoplasm
- Specific protocols can be used to further evaluate given conditions, including pituitary protocol studies, cranial nerve protocol studies, and special studies to evaluate seizures. These protocols will vary slightly on an institution-to-institution basis, and possibly even on a scanner-to-scanner basis within an institution.
MRI of the Orbits
- Can be performed without contrast, or with and without contrast. Contrast is typically administered when looking for signs of infection, a noninfectious inflammatory process, or a neoplasm
- Typically not performed in isolation; usually performed in addition to an MRI of the brain
Contraindications to MRI
MRI may be contraindicated for use in patients who have a metallic foreign body, a metallic prosthesis, or certain implanted medical devices such as most pacemakers and automated defibrillators. If a patient has an implanted medical device, it is critical to identify the exact device and confirm MRI compatibility. Renal failure is a contraindication to gadolinium administration.
Angiography
Angiography is defined as visual depiction of blood vessels. This can be accomplished by several means, including CT and MRI. Thin-section CT images of the head can be performed in the arterial phase after administration of intravenous contrast, resulting in a CT angiogram (CTA). If this is done slightly after the arterial phase, the venous structures can be evaluated as well, resulting in a CT venogram (CTV).
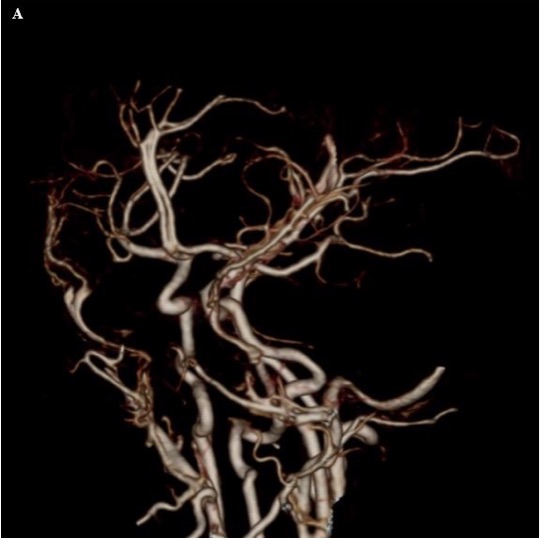
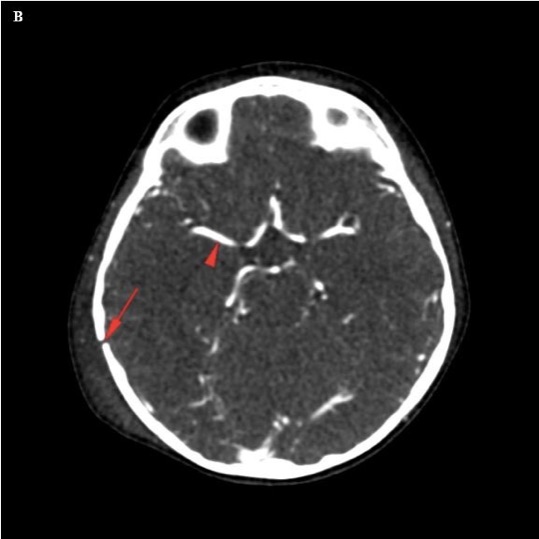
Figure 11. (A) Frontal oblique projection of a CT angiogram shows the intracranial vasculature, as well as the external carotid artery branches. (B) An axial source image used to create the CT angiogram shows a high-density appearance to the intracranial vasculature related to the iodinated contrast material (red arrowhead). A fracture is also seen (red arrow).
MR angiography (MRA) can also be performed, and typically does not require intravenous contrast, since it uses features of flowing blood to identify the vessels. The most common technique for performing this is known as time-of-flight angiography. If the direction of blood being evaluated is changed, it is possible to use time-of-flight imaging to perform an MR venogram (MRV) as well.
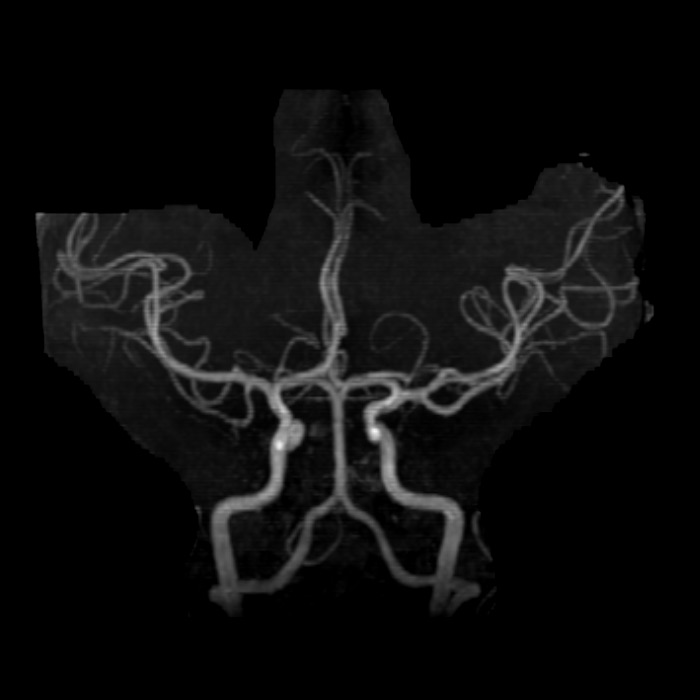
Figure 12. Frontal projection of an MR angiogram of the intracranial vasculature shows the anterior and posterior circulation. Cutouts can be performed to isolate specific vascular territories, looking for stenoses and aneurysms, among other pathologies.
Digital subtraction angiography (DSA) is an invasive angiographic technique that is considered the gold standard for cerebral vascular evaluation. The spatial resolution is much higher than CTA and MRA. DSA also has the benefit of a dynamic evaluation of blood flow through the arterial, capillary, and venous phases, and evaluation can be performed on a vessel-by-vessel basis. In DSA, X-ray fluoroscopy images are taken, intravenous (IV) dye (iodine-based) is injected, and follow-up images are taken fluoroscopically. The first images are digitally subtracted from the second, leading to a high-resolution view of the vascular structures. DSA is an invasive technique with an approximately 1% risk of stroke; however, the risks may be higher in children. DSA also uses more radiation than CTA and in children requires sedation.
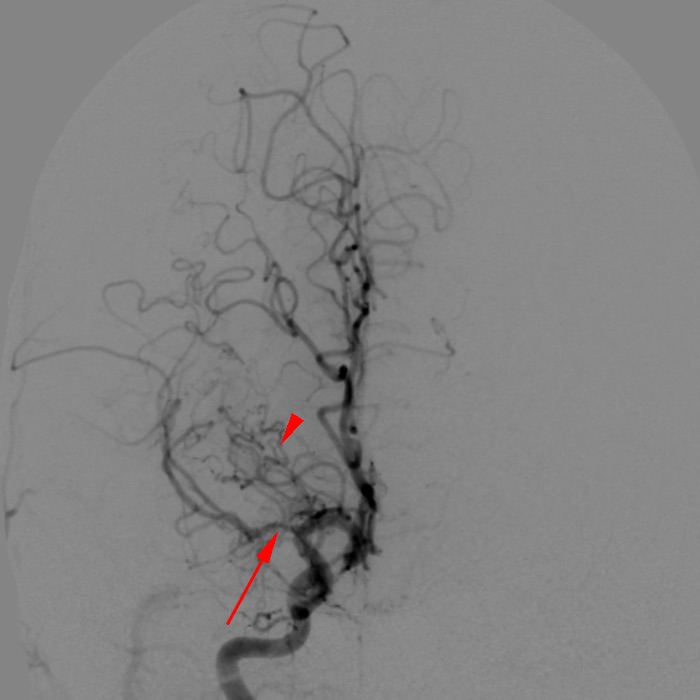
Figure 13. Frontal projection digital subtraction angiogram from a right internal carotid injection shows narrowing of the M1 segment of the right middle cerebral artery (red arrow), with numerous serpentine lenticulostriate collateral vessels in the region of the carotid terminus (red arrowhead), representing moyamoya vasculopathy. Moyamoya is a Japanese term meaning “hazy” or “ethereal,” and the disease gets this name from the appearance of these lenticulostriate collaterals. Note that moyamoya does not mean “puff-of-smoke,” despite the commonly stated mistranslation.
How to Order a CT or MRI
- Provide the reason for requesting the study. Beyond saying “rule out abscess,” state why you are worried about this by giving clinical context such as “fever, left-sided periorbital erythema and proptosis” or other signs and symptoms as appropriate.
- Confirm which eye(s) you are worried about.
- If possible, provide some information about the acuity of the problem, and whether it is an acquired or congenital issue. Acute left 6th cranial nerve palsy has different clinical, and therefore imaging, considerations than a congenital 6th nerve palsy. For instance, if imaging is necessary, MRI is likely the best initial study to evaluate a long-standing congenital 6th nerve palsy (note that imaging is typically not required for congenital forms of strabismus—this is for illustrative purposes only). CT may be able to provide rapid information about an acute finding to triage care, although MRI will eventually provide more information about the brain, brainstem, cranial nerves, skull base, and orbits.
- If following up on a finding from another study performed at another institution, state what was found in that other study (and if possible help obtain a copy of the outside study).
- If unsure about which test or variant is most appropriate, it is best to communicate directly with the radiologist or neuroradiologist prior to the study. This can help improve the diagnostic yield of the study by allowing the study to be optimized for the patient’s conditions. Similarly, after the study has been performed, communication with the radiologist regarding subtleties of the case can improve the interpretation beyond what could be achieved by either side in isolation.
Table 2. Imaging Indications and Recommended Diagnostic Studies
|
Imaging Indication
|
Diagnostic study
|
Additional Considerations
|
Acute vision loss
|
MRI of the brain and orbits with contrast
|
If post-traumatic, consider CT. If signs of infection, consider pre- and post-contrast imaging. Thorough clinical examination is necessary to identify nonorganic visual loss.
|
Strabismus/ Diplopia
|
MRI of the brain and orbits, with/without contrast; cranial nerve imaging
|
If patient has optic nerve head edema, add MR venography to look for venous sinus stenosis and/or thrombosis
|
Nystagmus
|
MRI of the brain with/without contrast
|
Clinical examination should identify pathology of anterior visual pathway (cataract), optic disc, or retinal pathology (lens, optic nerve, or retina).
|
Third nerve palsy
|
MRI brain and orbit with/without contrast
|
If post-trauma, perform CT; also consider angiographic evaluation (CTA or MRA), particularly in adults, to evaluate for posterior communicating artery aneurysm
|
Proptosis
|
MRI or CT of the orbit
|
Contrast if concerned for infectious, inflammatory, or neoplastic conditions
|
Poor visual fixation
|
MRI brain with/without contrast
|
Susceptibility weighted imaging to help identify signs of prior hemorrhage
|
Optic nerve edema
|
MRI brain, orbit with/without contrast; MRV
|
Bilateral, asymmetric optic nerve edema with unremarkable neuroimaging. Imaging requires a lumbar puncture to evaluate cerebrospinal fluid (CSF) composition to identify an infectious or neoplastic process. Opening pressure (≥ 280 mm CSF in children [250 mm CSF if the child is not sedated and not obese]) in a properly performed lumbar puncture with a normal neurologic examination and normal CSF composition is diagnostic of idiopathic intracranial hypertension. Optic neuritis is a clinical diagnosis; however, neuroimaging is helpful in identifying optic nerve enhancement, as well as white matter changes that may be associated with noninfectious inflammatory conditions such as multiple sclerosis or acute disseminated encephalomyelitis (ADEM).
|
Optic atrophy
|
MRI brain, orbit
|
Look for characteristic visual field defects associated with sellar/suprasellar lesions
|
CT, computed tomography; MRI, magnetic resonance imaging
References
- Goske MJ, Applegate KE, Boylan J, et al. The 'Image Gently' campaign: increasing CT radiation dose awareness through a national education and awareness program. Pediatr Radiol. 2008; 38:265–269.
- Pearce MS, Salotti JA, Little MP, et al. Radiation exposure from CT scans in childhood and subsequent risk of leukaemia and brain tumours: a retrospective cohort study. Lancet. 2012; 380:499-505.
- Blitz AM, Choudhri AF, Chonka ZD, et al. Anatomic considerations, nomenclature, and advanced cross-sectional imaging techniques for visualization of the cranial nerve segments by MR imaging. Neuroimaging Clin N Am. 2014; 24:1–15.
- Blitz AM, Macedo LL, Chonka ZD, et al. High-resolution CISS MR imaging with and without contrast for evaluation of the upper cranial nerves: segmental anatomy and selected pathologic conditions of the cisternal through extraforaminal segments. Neuroimaging Clin N Am. 2014; 24:17–34.
- Choudhri AF, Chin EM, Blitz AM, Gandhi D. Diffusion tensor imaging of cerebral white matter: technique, anatomy, and pathologic patterns. Radiol Clin North Am. 2014; 52:413–425.
- Pillai J, Zaca D, Choudhri A. Clinical impact of integrated physiologic brain tumor imaging. Technol Cancer Res Treat. 2010; 9:359–380.
- Silson EH, Chan AW, Reynolds RC, Kravitz DJ, Baker CI. A retinotopic basis for the division of high-level scene processing between lateral and ventral human occipitotemporal cortex. J Neurosci. 2015; 35:11921–11935.
Suggested Readings
Basics of Orbital Imaging
- Meltzer DE. Orbital imaging: a pattern-based approach. Radiol Clin North Am. 2015; 53:37–80.
Radiation Safety/Image Gently
- Goske MJ, Applegate KE, Boylan J, et al. The ‘Image Gently’ campaign: increasing CT radiation dose awareness through a national education and awareness program. Pediatr Radiol. 2008; 38(3):265–269.
- Pearce MS, Salotti JA, Little MP, et al. Radiation exposure from CT scans in childhood and subsequent risk of leukaemia and brain tumours: a retrospective cohort study. Lancet. 2012; 380(9840):499-505. doi: 10.1016/S0140-6736(12)60815-0. Epub 2012 Jun 7.
- Mathews JD, Forsythe AV, Brady Z, et al. Cancer risk in 680,000 people exposed to computed tomography scans in childhood or adolescence: data linkage study of 11 million Australians. BMJ. 2013; 346:f2360. doi: 10.1136/bmj.f2360.
Cranial Nerve Imaging
- Blitz AM, Choudhri AF, Chonka ZD, et al. Anatomic considerations, nomenclature, and advanced cross-sectional imaging techniques for visualization of the cranial nerve segments by MR imaging. Neuroimaging Clin N Am. 2014; 24(1):1–15.
- Blitz AM, Macedo LL, Chonka ZD, et al. High-resolution CISS MR imaging with and without contrast for evaluation of the upper cranial nerves: segmental anatomy and selected pathologic conditions of the cisternal through extraforaminal segments. Neuroimaging Clin N Am. 2014; 24(1):17–34.
Demyelinating Conditions
- Miller TR, Mohan S, Choudhri AF, Gandhi D, Jindal G. Advances in multiple sclerosis and its variants. Radiol Clin North Am. 2014; 52(2):321–336.
- Verhey LH, Shroff M, Banwell B. Pediatric multiple sclerosis: pathobiological, clinical, and magnetic resonance imaging features. Neuroimaging Clin N Am. 2013; 23(2):227–243.
- Makhani N, Bigi S, Banwell B, Shroff M. Diagnosing neuromyelitis optica. Neuroimaging Clin N Am. 2013; 23(2):279–291.
- Verhey LH, Shroff M, Banwell B. Pediatric multiple sclerosis: pathobiological, clinical, and magnetic resonance imaging features. Neuroimaging Clin N Am. 2013; 23(2):227–243.
- Thomas T, Branson HM. Childhood transverse myelitis and its mimics. Neuroimaging Clin N Am. 2013; 23(2):267–278.
- Moharir M, Shroff M, Benseler SM. Childhood central nervous system vasculitis. Neuroimaging Clin N Am. 2013; 23(2):293–308.
Advanced Techniques
- Choudhri AF, Chin EM, Blitz AM, Gandhi D. Diffusion tensor imaging of cerebral white matter. Radiol North Am. 2014:52(2):413–425.
- Pillai JJ, Zaca D, Choudhri A. Clinical impact of integrated physiologic brain tumor imaging. Technol Cancer Res Treat. 2010; 9(4):359–380.
Opening Pressure Evaluation in Pseudotumor Cerebri
- Friedman DI, Liu GT, Digre KB. Revised diagnostic criteria for the pseudotumor cerebri syndrome in adults and children. Neurology. 2013; 81:1159–1165. doi:10.1212/WNL.0b013e3182a55f17.